TEN TRILLION CELLS WALKED INTO A BAR
by PAUL INGRAHAM
Paul Ingraham is a writer and Registered
Massage Therapist in downtown Vancouver. At SaveYourself.ca,
Mr. Ingraham also waxes poetic and scientific about musculoskeletal
health care, publishing dozens of unusual
essays, handy tips, and advanced patient tutorials about
common injuries and pain problems like muscle
strain, low
back pain and neck
pain, runners injuries like iliotibial
band syndrome, and medically neglected problems like muscle
knots and trigger points.
You
are a colony of (at least) ten trillion cells.1 That
is what a human being is -- a very large social gathering of
cells. Ten trillion is a conservative estimate, but it is one
heck of a lot of cells. That is about 200,000 times more cells
in a single person than there are people on planet Earth. That’s
the sort of number that you really can’t get your head
around, even if you have a very big head.
SO
HOW DO TEN TRILLION CELLS WALK INTO A BAR?
How
did they walk, I mean? Or even stand up? It’s a bit of
a trick for ten trillion cells to do that. Individually, they
certainly couldn’t pull it off. A single cell would have
trouble walking six inches for a hot date with another single
cell.2
It’s
a cooperative effort, obviously. There are probably many committees,
subcommittees and review panels involved. But, in spite of the
complexity and bureaucracy, the end product -- walking -- is
efficient. Walking is so efficient, in fact, that it constitutes
one of the great mysteries of how people work. We still can’t
make a bipedal robot that can walk, not like us anyway -- “we,”
meaning geniuses at MIT studying robotics. We humans simply
don’t understand the details of our own locomotion.
But
we do have some idea how we at least stay upright. Even I understand
it. This superficially simple thing of rising up to a height
of six feet or more is an impressive feat for a bunch of cells
who are, individually, no taller than a coffee stain. But together
they pull it off, and that basic accomplishment is what I’ll
focus on here. This is stuff that cells probably learn in cell
kindergarten.
YOU
AREN'T STACKED
Contrary
to popular opinion, bones are not stacked on each other like
bricks. We do not really rest on our joints as much as you might
think, nor in the way you might think. There is compression
and friction in joints due to gravity, but this is not the supportive
principle by which we manage to get upright each morning and
stay that way.
Vertebrae
in particular are not really made for support. We are one of
the few creatures on Earth with an upright spine, the odd animal
out; all other vertebrates on Earth feature a horizontal spine,
which is much more obviously not built for bearing weight by
stacking. In fact, the spine we have is really not particularly
well-constructed for verticality. It’s as though we borrowed
a tool used by other species for hammering nails, and decided,
“Let’s use this for screwing in lightbulbs.”
It’s a bit queer, really.
In
fact, rather than being stacked, we are held together and upright
by muscles. Bare skeletons, as a general rule, fall over very
easily. In the living body, even when we think that we are completely
relaxed, our muscles are actually sustaining a constant level
of tension -- called “resting tone” — that
holds joints together.3 When we are anaesthetized,
surgeons must be cautious not to dislocate joints,4
because they become quite loose. This constant tension is what
we really stand on -- not bone resting on bone.
This
idea, in which the rigid elements of a system float in a continuous
tension network, was called “tensegrity” (tension/integrity)
by Buckminster Fuller. For a long time the ideas were more widely
known among architects than biologists,5 but “tensegrity
biomechanics” and “biotensegrity” are slowly
coming into their own.
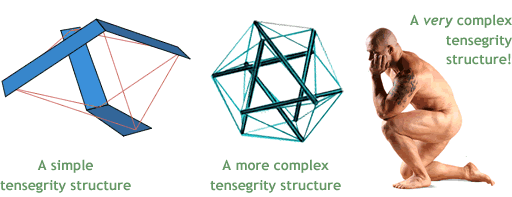
Bones
float in muscle, functioning more like “spacers”
than bricks. They provide rigidity for leverage and serve as
foundations for complex arrangements of high-tension wires (muscles
and tendons). We are pulled upright, and held upright, in much
the same way a circus tent pole is erected and held upright
-- not because it is resting on itself, but because it is being
pulled equally in all directions by ropes. Unlike a circus tent
pole, we actually need to move around, so this arrangement is
extremely dynamic and active, constantly at work even when we
are sitting.
There
is one other major principle that keeps us upright: hydrostatic
pressure.
WE
ARE MOSTLY BAGS OF WATER
Once
again, bones are of secondary importance to another more important
substance: soft connective tissue. Some aliens on an episode
of Star Trek: The Next Generation referred to humans as “ugly
bags of mostly water.” And right they were, at least about
the bags and the water. Ugly depends on which bag of water we’re
talking about (but let’s not go there).
The
point the aliens were trying to make was that humans are mostly
water -- and everyone knows that, right? More specifically,
and less widely known, is that our water is contained in flexible
membranes (bags). The bag, or sack is made of our connective
tissue, intricate layers of a substance somewhat like Saran
Wrap that literally holds us together. We have more connective
tissue than anything else. 6
The
water (hydro) inside of us is under constant (static) pressure
-- hydrostatic pressure. The bag is tight. This is exactly like
putting a tight elastic band around a water balloon: it squishes
it into a more elongated shape. If you were to put several rubber
bands in a row around a water balloon, it would start to look
more like a tube than a balloon. In fact, it might start to
resemble, say, a leg. If only it could balance itself, this
“balloon leg” could stand upright -- thanks to the
pressure of the water inside.
This
is entirely how plants stand up. Spinach has no spine, no bones
at all, but it still manages to stand up. Unless you don’t
water it, and then it wilts -- no water, no pressure, no standing
up. And of course there is one part of the human body, the male
human body specifically, that illustrates this principle --
perfectly.
TA
DA!
Our
ten trillion cells manage to walk into a bar by applying two
major physical principles: tensegrity and hydrostatic pressure.
Our cells build tough membranes to tightly surround compartments
of pressurized water, they make rigid bones to act as spacers
and points of leverage, and they arrange themselves in complex
systems of muscle tissue in order to literally pull us into
the vertical position and keep us there like a tent pole.
How
ten trillion cells order a tall cold one and generate bad pick-up
lines is a completely different mystery altogether.
Notes
1. Actually, this number is in the middle of a stunning range
of possibilities suggested by experts over the last century.
The highest estimate I’ve ever seen, published in 2003
in the popular but credible book A short history of nearly everything
, by Bill Bryson, was in the quadrillions — that’s
right, quadrillions, as in thousands of trillions! In 2006 in
National Geographic, citing various experts, Joel Achenbach
reports that microbes living in our bodies outnumber our own
cells 10 to 1 and there are a hundred trillion microorganisms
in the intestines alone — so those figures would also
put the total for the whole organism well into the quadrillions.
At the other extreme, estimates of the number of cells we have
range as low as tens of billions. But, regardless, we have a
lot of cells!
2. If you do the math, “walking” six inches for
a cell is like us walking about a mile — not too far for
a hot date.
3. There is just one joint in the body that can maintain its
integrity after complete muscular dissection: the hip joint.
The socket of that joint is so deep and perfectly fitted to
the ball of the femur, and the capsule of ligaments around it
so sturdy and air tight, that it is held nicely in place by
“suction.” However, cut a tiny hole in the capsule
with a scalpel, and the joint immediately dislocates!
4.
Casey et al. BMJ. 1995.
5.
“Tensegrity.” Wikipedia.com.
6. We really do have a lot of connective tissue, but connective
tissue also includes some surprising and counterintuitive tissues
like blood and fat.